Example Experiment: Detecting p53 Activation in COS-7 Cells Following Hydroxyurea Treatment
Background
Accurate quantification of protein expression and/or post-translational modifications is important for advancing both basic and translational research. Given the flexibility, reproducibility, and higher throughput of the In‑Cell Western™ Assay, it offers a convenient alternative to Western blotting and is a powerful platform for meaningful in situ analyses. The In-Cell Western microplate format can be used to analyze:
- Protein phosphorylation and signaling (1 - 3)
- Off-target effects of drugs on signaling pathways (4)
- Timing and kinetics of signaling events (5, 6)
- Quantification of viral load (7 - 11)
- Genotoxicity assays (12, 13)
- Cell proliferation and apoptosis assays (14)
- Bacterial-induced epithelial signaling (15)
- Glycoprotein analysis (16, 17)
- Library screening (18 - 20)
- Screening of monoclonal antibody clones (21)
Introduction
P53 is a transcription factor that regulates the expression of numerous genes in response to stress stimuli (22). Many of these genes play important roles in apoptosis, senescence, cellular metabolism, the DNA damage response, and the oxidative stress response (23). In response to DNA damage, p53 governs the activation of specific gene expression programs to achieve the desired cellular outcomes through multiple layers of regulation (24). For example, p53 is phosphorylated at several sites by different protein kinases, each triggering a specific response (25). DNA damage stimulates p53 phosphorylation at Ser15 and Ser20, disrupting the interaction between p53 and the oncoprotein, MDM2, its negative regulator (26).
Understanding how new therapies impact this complex pathway is of great importance to the drug discovery field. For example, 50% of cancers lose p53 function as a result of mutations that produce single amino-acid substitutions in the core DNA-binding domain (27). In other cancers, wild-type p53 is targeted for degradation by cellular (MDM2) or viral (E6) oncoproteins (28, 29). As a result, many aggressive forms of cancers are resistant to traditional chemotherapy regimens owing to the lack of p53-dependent apoptosis (30). Therefore, developing cell-based assays that can identify compounds capable of exploiting the p53 pathway by either displaying synthetic lethality in cancers with p53 mutations or by looking for non-genotoxic activators of the p53 response, is of great importance. In the following example, we illustrate how the In‑Cell Western™ Assay can be utilized to quantify p53 activation after drug treatment in the p53-wildtype cell line, COS-7.
Experimental Design
p53 activation is monitored using an antibody that detects endogenous levels of p53 that have been phosphorylated on serine residue, 15. This antibody does not recognize p53 that has been phosphorylated on other sites. Phosphorylated p-53 (Ser15) levels are then normalized against total ERK1 protein levels. Hydroxyurea, a potent teratogen and anticancer agent, induces oxidative stress and activates a DNA damage response pathway (31, 32). Therefore, we investigated the effects of hydroxyurea on p53 activation in COS-7 cells by In-Cell Western.
Required Reagents
LI‑COR Reagents
-
IRDye® 800CW Goat anti-Rabbit Secondary Antibody (LI-COR P/N 925-32211 or 926-32211)
-
IRDye 680RD Goat anti-Mouse Secondary Antibody (LI-COR P/N 925-68070 or 926-68070)
-
Intercept® (PBS) Blocking Buffer
Odyssey® Blocking Buffer was used in the original experiment and has been discontinued. Intercept Blocking Buffer is now available instead of Odyssey Blocking Buffer.
Additional Reagents
- 1X PBS wash buffer
- COS-7 cells (ATCC; CRL-1651)
- Tissue culture reagents (serum, DMEM, trypsin, 1X PBS)
- Black-sided 96-well or 384-well microplates with clear well bottoms
- Hydroxyurea (Sigma®, P/N H8627)
- Anti-phospho-p53 (Cell Signaling Technology, P/N 9286)
-
Normalization antibody: Anti-total ERK1 (Santa Cruz Biotechnology, P/N SC-94)
This has been discontinued. Suitable alternatives may be obtained from Cell Signaling Technology.
- 20% Tween® 20
- 37% formaldehyde
- 10% Triton® X-100
Prepare Cells
-
Allow COS-7 (ATCC; CRL-1651) cell growth in a T75 flask using standard tissue culture procedures until ~80% confluency is achieved (~1.5 x 107 cells; DMEM, 10% FBS; Gibco®).
-
Remove growth media and wash cells with sterile 1X PBS (room temperature (RT)).
-
Add trypsin and incubate 3-5 minutes at 37 °C to displace cells.
-
Neutralize displaced cells with culture media and pellet by centrifugation (500 x g).
-
Remove supernatant and disrupt the cell pellet manually by hand-tapping the collection tube.
To maintain cell integrity, do not pipet or vortex during pellet disruption.
-
Resuspend cells in 20 mL of complete media and count cells using a hemocytometer.
-
Reconstitute and dilute cells with complete media to a concentration of 100,000 cells/mL.
-
Manually mix the cell suspension thoroughly.
-
Under sterile conditions, dispense 200 µL of the cell suspension per well in a 96-well plate (20,000 cells plated per well).
-
Incubate cells at 37 °C with 5% CO2 and monitor cell density until ~80% confluency is achieved.
Treat Cells
- Warm serum-free media (DMEM, Gibco) to 37 °C.
- Remove complete media from plate wells by aspiration or manual displacement.
- Add either serum-free media for resting cells (mock) or serum-free media with serial concentrations of Hydroxyurea ranging 0.04 - 20 mM for activated cells. Add 100 µL of resting or activation media per well.
- Incubate at 37 °C with 5% CO2 overnight (16 - 24 hours).
Fix and Permeabilize Cells
Fix Cells
-
Prepare fresh Fixing Solution as follows:
1X PBS 45 mL 37% Formaldehyde 5 mL 3.7% Formaldehyde 50 mL -
When incubation period is complete, carefully remove activation media manually or by aspiration to avoid detaching the cells.
-
Using a multi-channel pipettor, add 150 µL of fresh Fixing Solution (RT). Add the solution by pipetting down the sides of the wells carefully to avoid detaching the cells from the well bottom.
-
Allow incubation on bench top for 20 minutes at RT with no shaking.
Permeabilize Cells
-
Prepare Triton® Washing Solution as follows:
1X PBS 495 mL 10% Triton X-100 5 mL 1X PBS + 0.1% Triton X-100 500 mL -
Remove Fixing Solution to an appropriate waste container (contains formaldehyde).
-
Using a multi-channel pipettor, add 200 µL Triton Washing Solution (RT). Add the solution down the sides of the wells carefully to avoid detaching the cells.
-
Allow plate to shake on a rotator for 5 minutes at RT.
-
Repeat washing steps 4 more times, removing wash manually each time.
Do not allow cells to become dry during washing. Immediately add the next wash after manual disposal.
Block Cells
- Using a multi-channel pipettor, add 150 µL of Intercept® Blocking Buffer to each well. Add the solution by pipetting down the sides of the wells carefully to avoid detaching the cells.
- Allow blocking for 1.5 hours at RT with moderate shaking on a plate shaker.
Primary Antibodies
Dilute Primary Antibodies
-
Dilute the two primary antibodies in Intercept Blocking Buffer. Combine the following antibodies for phospho-p53 target analysis, using total ERK1 for normalization:
Phospho-p53; mouse (1:400 dilution in the combined solution; Cell Signaling Technology, P/N 9286)
Total ERK1; rabbit (1:100 dilution in the combined solution; Santa Cruz Biotechnology, P/N SC-94)
-
Mix the primary antibody solution thoroughly before adding to wells.
Incubate with Primary Antibodies
- Remove blocking buffer from the blocking step and add 50 µL of the desired primary antibody or antibodies in Intercept Blocking Buffer to cover the bottom of each well.
- Make sure to include control wells without primary antibody to serve as a source for background well intensity. Only add 50 µL of Intercept Blocking Buffer to control wells.
- Incubate with primary antibody overnight with gentle shaking at 4 °C.
Wash
-
Prepare Tween® Washing Solution as follows:
1X PBS 995 mL 20% Tween 20 5 mL 1X PBS + 0.1% Tween 20 1000 mL -
Remove primary antibody solution.
-
Using a multi-channel pipettor, add 200 µL Tween Washing Solution (RT). Add solution down the sides of the wells carefully to avoid detaching the cells from the well bottom.
-
Allow wash to shake on plate shaker for 5 minutes at RT.
-
Repeat washing steps 4 more times.
Secondary Antibodies
Dilute Secondary Antibodies
-
Dilute the fluorescently-labeled secondary antibodies in Intercept® Blocking Buffer as specified below. To lower background, add Tween 20 to the diluted antibody to a final concentration of 0.2%. Recommended dilution range is 1:200 – 1:1,200.
Goat anti-Mouse IRDye® 680RD (1:800 dilution in the combined solution)
Goat anti-Rabbit IRDye 800CW (1:800 dilution in the combined solution)
Minimize exposure of the antibody vials to light.
-
Mix the antibody solutions and add 50 µL of the secondary antibody solution to each well.
Incubate with Secondary Antibodies
- Incubate for 60 minutes with gentle shaking at RT. Protect plate from light during incubation.
Wash
- Remove secondary antibody solution.
- Using a multi-channel pipettor, add 200 µL of Tween Washing Solution at RT (Wash). Add solution down the sides of the wells carefully to avoid detaching the cells from the well bottom.
- Allow wash to incubate on a plate shaker for 5 minutes at RT.
- Repeat washing steps 4 more times. Protect plate from light during washing.
Image
- After final wash, remove wash solution completely from wells. Turn the plate upside down and tap or blot gently on paper towels to remove traces of wash buffer. For best results, scan plate immediately; plates may also be stored at 4 °C for several weeks (sealed and protected from light).
- Before plate scanning, clean the bottom plate surface and the Odyssey® Imager scanning bed (if applicable) with moist, lint-free tissue to avoid any obstructions during scanning.
- Scan plate with detection in both 700 and 800 nm channels.
Suggested Scan Settings
All settings may require adjustment for optimal data quality. Higher resolutions or scan qualities can be used, but the scan time will increase.
Instrument | Resolution | Scan Quality | Intensity Setting (700 nm) | Intensity Setting (800 nm) |
Odyssey Classic | 169 µM | lowest | 5 | 5 |
Odyssey DLx | 169 µM | lowest | Auto Mode | Auto Mode |
Experimental Results
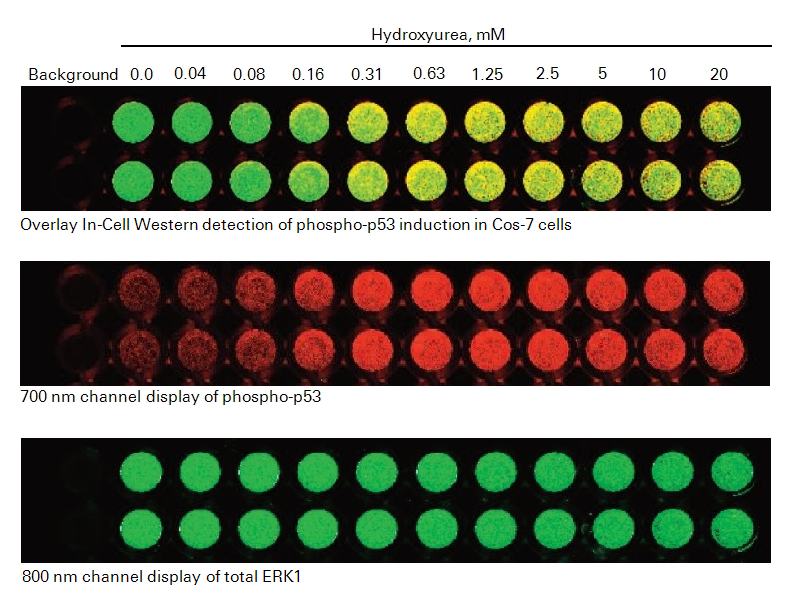
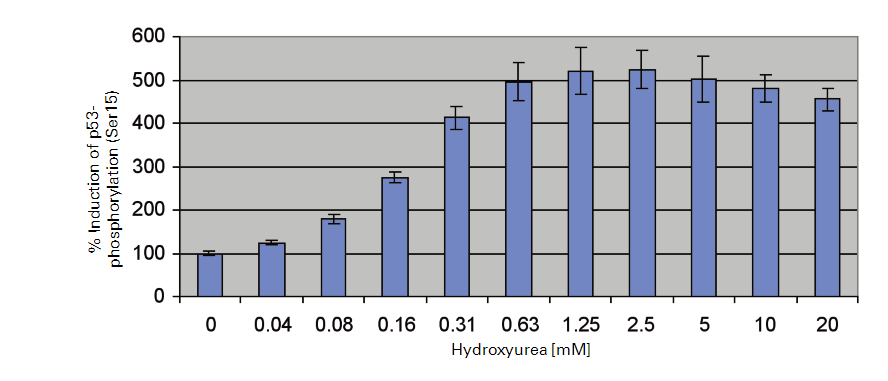
References
1. Chen, H., Kovar, J., Sissons, S., Cox, K., Matter, W., Chadwell, F., Luan, P., Vlahos, C. J., Schutz-Geschwender, A., and Olive, D. M. (2005) A cell-based immunocytochemical assay for monitoring kinase signaling pathways and drug efficacy. Analytical biochemistry 338, 136-142
2. Aguilar, H. N., Zielnik, B., Tracey, C. N., and Mitchell, B. F. (2010) Quantification of rapid Myosin regulatory light chain phosphorylation using high-throughput in-cell Western assays: comparison to Western immunoblots. PLoS One 5, e9965
3. Wong, S. K. (2004) A 384-well cell-based phospho-ERK assay for dopamine D2 and D3 receptors. Analytical biochemistry 333, 265-272
4. Kumar, N., Afeyan, R., Kim, H. D., and Lauffenburger, D. A. (2008) Multipathway model enables prediction of kinase inhibitor cross-talk effects on migration of Her2-overexpressing mammary epithelial cells. Mol Pharmacol 73, 1668-1678
5. Hannoush, R. N. (2008) Kinetics of Wnt-driven beta-catenin stabilization revealed by quantitative and temporal imaging. PLoS One 3, e3498
6. Chen, W. W., Schoeberl, B., Jasper, P. J., Niepel, M., Nielsen, U. B., Lauffenburger, D. A., and Sorger, P. K. (2009) Input-output behavior of ErbB signaling pathways as revealed by a mass action model trained against dynamic data. Mol Syst Biol 5, 239
7. Counihan, N. A., Daniel, L. M., Chojnacki, J., and Anderson, D. A. (2006) Infrared fluorescent immunofocus assay (IR-FIFA) for the quantitation of non-cytopathic and minimally cytopathic viruses. J Virol Methods 133, 62-69
8. Lin, Y. C., Li, J., Irwin, C. R., Jenkins, H., DeLange, L., and Evans, D. H. (2008) Vaccinia virus DNA ligase recruits cellular topoisomerase II to sites of viral replication and assembly. J Virol 82, 5922-5932
9. Weldon, S. K., Mischnick, S. L., Urlacher, T. M., and Ambroz, K. L. (2010) Quantitation of virus using laser-based scanning of near-infrared fluorophores replaces manual plate reading in a virus titration assay. J Virol Methods 168, 57-62
10. Lopez, T., Silva-Ayala, D., Lopez, S., and Arias, C. F. (2012) Methods suitable for high-throughput screening of siRNAs and other chemical compounds with the potential to inhibit rotavirus replication. J Virol Methods 179, 242-249
11. Wan, Y., Zhou, Z., Yang, Y., Wang, J., and Hung, T. (2010) Application of an In-Cell Western assay for measurement of influenza A virus replication. J Virol Methods 169, 359-364
12. Jamin, E. L., Riu, A., Douki, T., Debrauwer, L., Cravedi, J. P., Zalko, D., and Audebert, M. (2013) Combined genotoxic effects of a polycyclic aromatic hydrocarbon (B(a)P) and an heterocyclic amine (PhIP) in relation to colorectal carcinogenesis. PLoS One 8, e58591
13. Khoury, L., Zalko, D., and Audebert, M. (2013) Validation of high-throughput genotoxicity assay screening using gammaH2AX in-cell western assay on HepG2 cells. Environ Mol Mutagen 54, 737-746
14. Godin-Heymann, N., Ulkus, L., Brannigan, B. W., McDermott, U., Lamb, J., Maheswaran, S., Settleman, J., and Haber, D. A. (2008) The T790M "gatekeeper" mutation in EGFR mediates resistance to low concentrations of an irreversible EGFR inhibitor. Mol Cancer Ther 7, 874-879
15. Du, Y., Danjo, K., Robinson, P. A., and Crabtree, J. E. (2007) In-Cell Western analysis of Helicobacter pylori-induced phosphorylation of extracellular-signal related kinase via the transactivation of the epidermal growth factor receptor. Microbes Infect 9, 838-846
16. McInerney, M. P., Pan, Y., Short, J. L., and Nicolazzo, J. A. (2017) Development and Validation of an In-Cell Western for Quantifying P-Glycoprotein Expression in Human Brain Microvascular Endothelial (hCMEC/D3) Cells. J Pharm Sci 106, 2614-2624
17. Urlacher T, Xing K, Cheung L et al (2013) Glycoprotein applications using near-infrared detection. Poster presentation, Experimental Biology
18. Guo, K., Shelat, A. A., Guy, R. K., and Kastan, M. B. (2014) Development of a cell-based, high-throughput screening assay for ATM kinase inhibitors. J Biomol Screen 19, 538-546
19. Hoffman, G. R., Moerke, N. J., Hsia, M., Shamu, C. E., and Blenis, J. (2010) A high-throughput, cell-based screening method for siRNA and small molecule inhibitors of mTORC1 signaling using the In Cell Western technique. Assay Drug Dev Technol 8, 186-199
20. Schnaiter, S., Furst, B., Neu, J., Waczek, F., Orfi, L., Keri, G., Huber, L. A., and Wunderlich, W. (2014) Screening for MAPK modulators using an in-cell western assay. Methods Mol Biol 1120, 121-129
21. Daftarian, M. P., Vosoughi, A., and Lemmon, V. (2014) Gene-based vaccination and screening methods to develop monoclonal antibodies. Methods Mol Biol 1121, 337-346
22. Chang, G. S., Chen, X. A., Park, B., Rhee, H. S., Li, P., Han, K. H., Mishra, T., Chan-Salis, K. Y., Li, Y., Hardison, R. C., Wang, Y., and Pugh, B. F. (2014) A comprehensive and high-resolution genome-wide response of p53 to stress. Cell Rep 8, 514-527
23. Aylon, Y., and Oren, M. (2007) Living with p53, dying of p53. Cell 130, 597-600
24. Hafner, A., Bulyk, M. L., Jambhekar, A., and Lahav, G. (2019) The multiple mechanisms that regulate p53 activity and cell fate. Nat Rev Mol Cell Biol 20, 199-210
25. Milczarek, G. J., Martinez, J., and Bowden, G. T. (1997) p53 Phosphorylation: biochemical and functional consequences. Life Sci 60, 1-11
26. Shieh, S. Y., Ikeda, M., Taya, Y., and Prives, C. (1997) DNA damage-induced phosphorylation of p53 alleviates inhibition by MDM2. Cell 91, 325-334
27. Olivier, M., Eeles, R., Hollstein, M., Khan, M. A., Harris, C. C., and Hainaut, P. (2002) The IARC TP53 database: new online mutation analysis and recommendations to users. Hum Mutat 19, 607-614
28. Momand, J., Zambetti, G. P., Olson, D. C., George, D., and Levine, A. J. (1992) The mdm-2 oncogene product forms a complex with the p53 protein and inhibits p53-mediated transactivation. Cell 69, 1237-1245
29. Scheffner, M., Werness, B. A., Huibregtse, J. M., Levine, A. J., and Howley, P. M. (1990) The E6 oncoprotein encoded by human papillomavirus types 16 and 18 promotes the degradation of p53. Cell 63, 1129-1136
30. Hientz, K., Mohr, A., Bhakta-Guha, D., and Efferth, T. (2017) The role of p53 in cancer drug resistance and targeted chemotherapy. Oncotarget 8, 8921-8946
31. Nayak, B. K., and Das, G. M. (2002) Stabilization of p53 and transactivation of its target genes in response to replication blockade. Oncogene 21, 7226-7229
32. Ho, C. C., Siu, W. Y., Lau, A., Chan, W. M., Arooz, T., and Poon, R. Y. (2006) Stalled replication induces p53 accumulation through distinct mechanisms from DNA damage checkpoint pathways. Cancer Res 66, 2233-2241